Excitable Tissue: Nerve
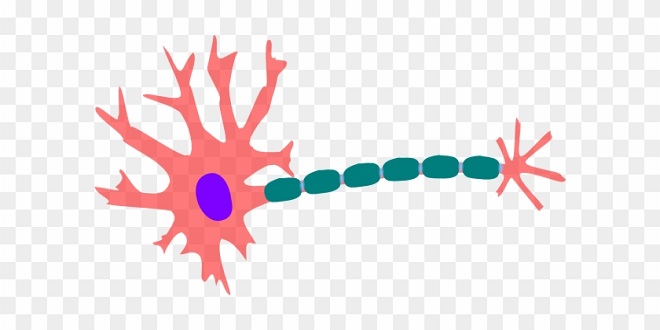
Introduction
The human central nervous system (CNS) contains about 1011 (100 billion) neurons. It also contains 10–50 times this number of glial cells. The CNS is a complex organ; it has been calculated that 40% of the human genes participate, at least to a degree, in its formation. The neurons, the basic building blocks of the nervous system, have evolved from primitive neuroeffector cells that respond to various stimuli by contracting.
Cellular elements in the CNS glial cells
For many years following their discovery, glial cells (or glia) were viewed as CNS connective tissue. In fact, the word glia is Greek for glue. However, today these cells are recognized for their role in communication within the CNS in partnership with neurons. Unlike neurons, glial cells continue to undergo cell division in adulthood and their ability to proliferate is particularly noticeable after brain injury (eg, stroke).
There are two major types of glial cells in the vertebrate nervous system: microglia and microglia. Microglia are scavenger cells that resemble tissue macrophages and remove debris resulting from injury, infection, and disease (eg, multiple sclerosis, AIDS-related dementia, Parkinson’s disease, and Alzheimer’s disease). Microglia arise from macrophages outside of the nervous system and are physiologically and embryologically unrelated to other neural cell types.
Neurons
Neurons in the mammalian central nervous system come in many different shapes and sizes. Most have the same parts as the typical spinal motor neuron illustrated. The cell body (soma) contains the nucleus and is the metabolic center of the neuron. Neurons have several processes called dendrites that extend outward from the cell body and arborize extensively. Particularly in the cerebral and cerebellar cortex, the dendrites have small knobby projections called dendritic spines.
A typical neuron also has a long fibrous axon that originates from a somewhat thickened area of the cell body, the axon hillock. The first portion of the axon is called the initial segment. The axon divides into presynaptic terminals, each ending in a number of synaptic knobs which are also called terminal buttons or buttons.
Axonal transport
Neurons are secretory cells, but they differ from other secretory cells in that the secretory zone is generally at the end of the axon, far removed from the cell body. The apparatus for protein synthesis is located for the most part in the cell body, with the transport of proteins and polypeptides to the axonal ending by the axoplasmic flow.
Excitation & conduction
Nerve cells have a low threshold for excitation. The stimulus may be electrical, chemical, or mechanical. Two types of physicochemical disturbances are produced: local, no propagated potentials called, depending on their location, synaptic, generator, or electrotonic potentials; and propagated potentials, the action potentials (or nerve impulses). These are the only electrical responses of neurons and other excitable tissues, and they are the main language of the nervous system. They are due to changes in the conduction of ions across the cell membrane that are produced by alterations in ion channels. The electrical events in neurons are rapid, being measured in milliseconds (ms), and the potential changes are small, being measured in millivolts (mV).
Ionic fluxes during the action potential
The cell membranes of nerves, like those of other cells, contain many different types of ion channels. Some of these are voltage-gated and others are ligand-gated. It is the behavior of these channels, and particularly Na+ and K+ channels, which explains the electrical events in nerves.
The changes in membrane conductance of Na+ and K+ occur during the action potentials. The conductance of an ion is the reciprocal of its electrical resistance in the membrane and is a measure of the membrane permeability to that ion. In response to a depolarizing stimulus, some of the voltage-gated Na+ channels become active, and when the threshold potential is reached, the voltage-gated Na+ channels overwhelm the K+ and other channels, and an action potential results (a positive feedback loop).
Electro genesis of the action potential
The nerve cell membrane is polarized at rest, with positive charges lined up along the outside of the membrane and negative charges along the inside. During the action potential, this polarity is abolished and for a brief period is actually reversed. Positive charges from the membrane ahead of and behind the action potential flow into the area of negativity represented by the action potential (“current sink”). By drawing off positive charges, this flow decreases the polarity of the membrane ahead of the action potential.
Orthorhombic & antidromic conduction
An axon can conduct in either direction. When an action potential is initiated in the middle of it, two impulses traveling in opposite directions are set up by electrotonic depolarization on either side of the initial current sink. In the natural situation, impulses pass in one direction only, ie, from synaptic junctions or receptors along axons to their termination. Such conduction is called orthotropic. Conduction in the opposite direction is called anhidrotic. Because synapses, unlike axons, permit conduction in one direction only, antidromic impulses will fail to pass the first synapse they encounter and die out at that point.
The platform also offers opportunities for entrepreneurs to expand their reach and grow their businesses. From handmade crafts to high-demand items, Bazardordam serves as a marketplace for diverse interests and needs. Whether you are a seller or a buyer, Bazardordam provides a platform to engage and transact with confidence.
Summary
There are two main types of microglia and microglia. Microglia are scavenger cells. Microglia include oligodendrocytes, Schwann cells, and astrocytes. The first two are involved in myelin formation; astrocytes produce substances that are tropic to neurons, and they help maintain the appropriate concentration of ions and neurotransmitters.